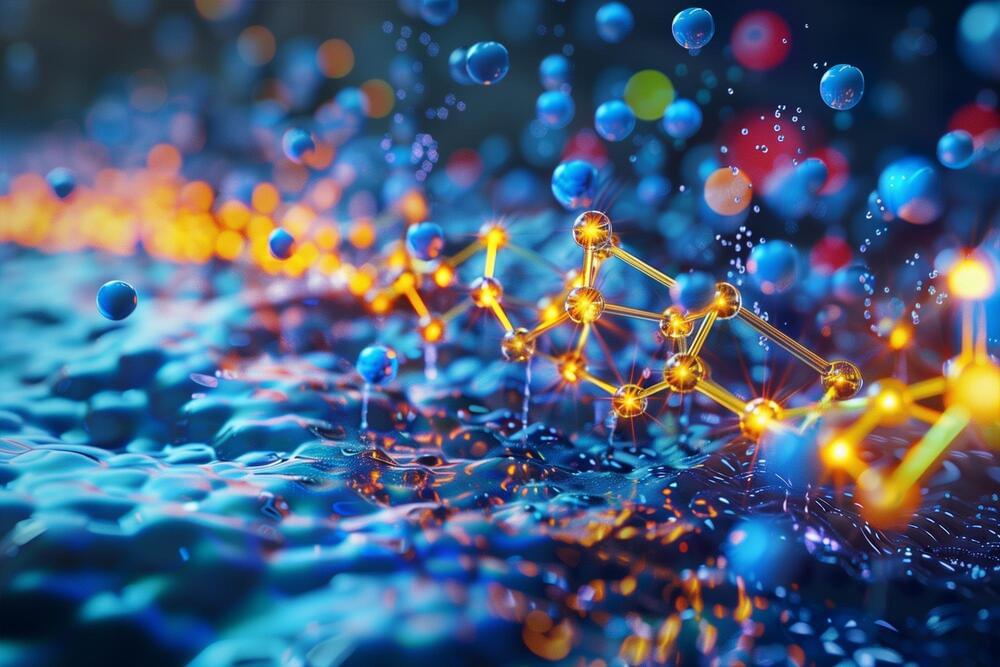
EPFL researchers have discovered that nanoscale devices harnessing the hydroelectric effect can harvest electricity from the evaporation of fluids with higher ion concentrations than purified water, revealing a vast untapped energy potential.
Evaporation is a natural process so ubiquitous that most of us take it for granted. In fact, roughly half of the solar energy that reaches the earth drives evaporative processes. Since 2017, researchers have been working to harness the energy potential of evaporation via the hydrovol~aic (HV) effect, which allows electricity to be harvested when fluid is passed over the charged surface of a nanoscale device. Evaporation establishes a continuous flow within nanochannels inside these devices, which act as passive pumping mechanisms. This effect is also seen in the microcapillaries of plants, where water transport occurs thanks to a combination of capillary pressure and natural evaporation.
Although hydrovoltaic devices currently exist, there is very little functional understanding of the conditions and physical phenomena that govern HV energy production at the nanoscale. It’s an information gap that Giulia Tagliabue, head of the Laboratory of Nanoscience for Energy Technology (LNET) in the School of Engineering, and PhD student Tarique Anwar wanted to fill. They leveraged a combination of experiments and multiphysics modelling to characterize fluid flows, ion flows, and electrostatic effects due to solid-liquid interactions, with the goal of optimizing HV devices.