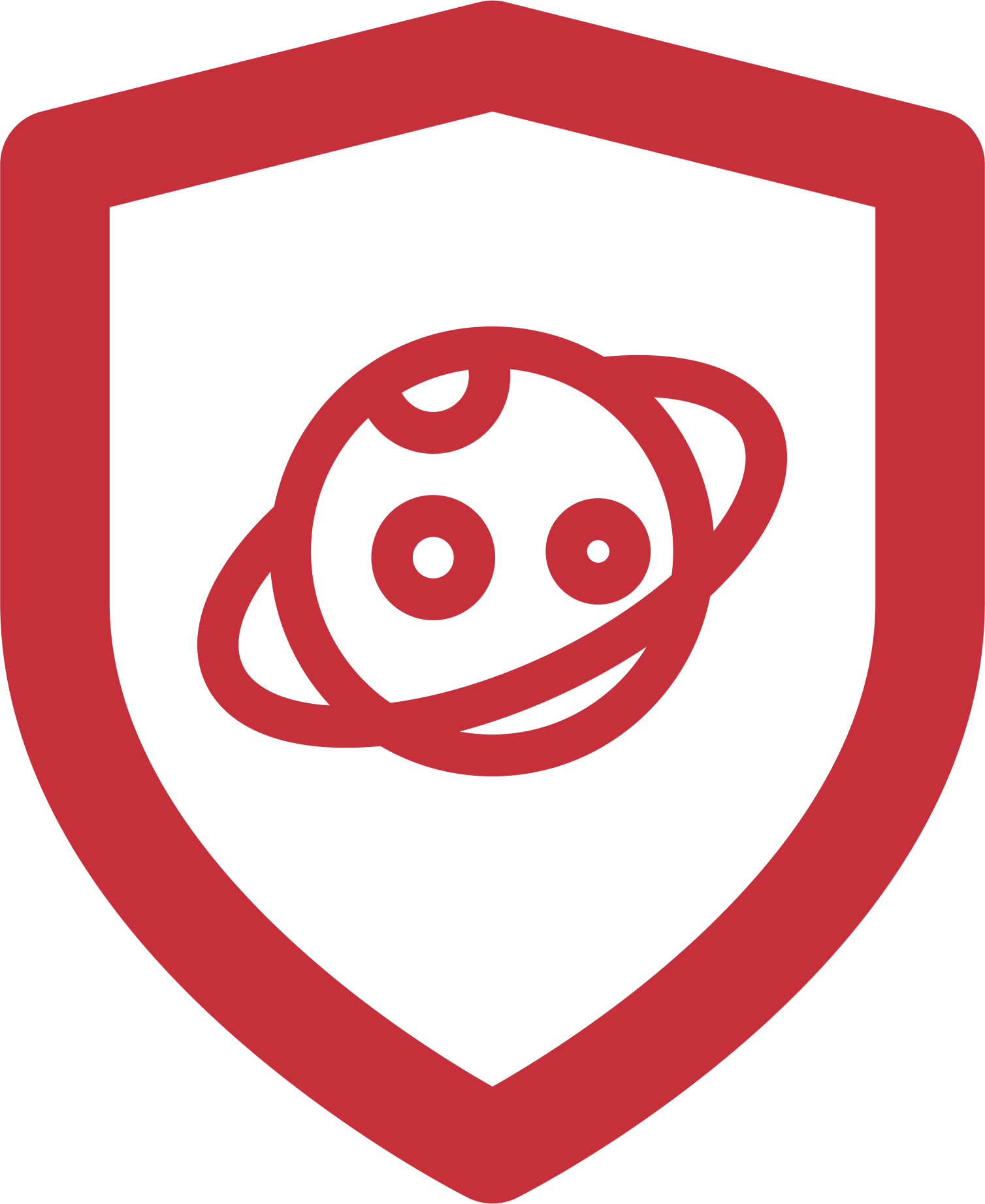
Lifeboat Foundation Space Habitats
By James Doehring and members of the Lifeboat Foundation Scientific Advisory Board. This is an ongoing program so you may submit suggestions to programs@lifeboat.com.
Overview
Establishing self-sufficient space habitats will serve as a backup plan for human civilization. A number of key milestones need to be reached before the long-term development of space is feasible, however. Improved access to space will catalyze the establishment of such habitats by allowing more frequent and less expensive flights beyond the atmosphere. Innovative, non-rocket methods of reaching orbit will enable more substantial progress in space.
Artificial ecosystems will need to be made as independent as possible to minimize the need for new resources. Better management of and access to resources from non-terrestrial bodies will allow astronauts to get the most out of what they do have. Finally, further countermeasures against the effects of space on health will be required to sustain human life in space.
The Lifeboat Foundation has begun design on Ark I, a self-sustaining space habitat. We support the efforts by SpaceX and others to make access to space more affordable. Likewise, we support the efforts of Bigelow Aerospace and others to develop habitable environments in space.
Improved Access to Space
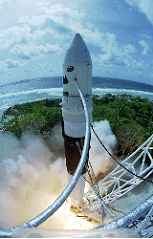
After more than fifty years of development, launch vehicles (rockets that reach space) still have severe limitations in what they can get into space. It appears extraordinarily difficult to increase the dimensions of a rocket’s payload volume, which restricts payloads to a certain size. For this reason, space structures have been built by assembling separate, smaller modules. Inflatable modules may increase this upper limit on module size, but fundamental limitations will always exist with launch vehicle technology.
Furthermore, the high cost of rocket launches prohibits all but large corporations, governmental agencies, and the wealthiest of tourists from reaching low Earth orbit. Improvements in the economics of launch vehicles can reduce the cost of accessing space in the short term, but radical non-rocket space launch technologies will be required in the long term.
Many such ideas have been proposed, but it’s difficult to meaningfully compare them and get a sense of what’s actually on the technology horizon. The best way to quantitatively assess these technologies is by using Technology Readiness Levels (TRLs). TRLs are used by NASA, the United States military, and many other agencies and companies worldwide. Typically there are nine levels, ranging from speculations on basic principles to full flight-tested status.
The system NASA uses can be summed up as follows:
- TRL 1 Basic principles observed and reported
- TRL 2 Technology concept and/or application formulated
- TRL 3 Analytical and experimental critical function and/or characteristic proof-of concept
- TRL 4 Component and/or breadboard validation in laboratory environment
- TRL 5 Component and/or breadboard validation in relevant environment
- TRL 6 System/subsystem model or prototype demonstration in a relevant environment (ground or space)
- TRL 7 System prototype demonstration in a space environment
- TRL 8 Actual system completed and “flight qualified” through test and demonstration (ground or space)
- TRL 9 Actual system “flight proven” through successful mission operations.
Below is a survey of existing ideas for reaching space without relying on rockets.
Space Gun TRL 6
Space guns are not intended to transport humans, but they may one day hurl cargo or robotic equipment into space. In a gun, all of the positive acceleration of a projectile takes place inside the barrel. The same is true of a space gun. Like with a bullet, this requires that a projectile undergo a tremendous amount of acceleration. It must accordingly be very rugged; a weak projectile would not survive intact. Nonetheless, the US Navy’s HARP Project launched a projectile to 180 kilometers-around the boundary of low Earth orbit. A robust projectile could be brought into a stable orbit by attaching a small rocket to it.
Space Plane TRL 6
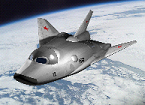
A version of a space plane called SpaceShipOne reached a 100 kilometer altitude on June 21, 2004. It was also the first privately-funded venture into space. While showing great promise for reaching suborbital trajectories, fully orbital space planes will likely suffer all the drawbacks of the Space Shuttle. Winged lifting bodies need to carry their own propellant and oxidizer when in space, and this requirement tends to lead to an aerodynamic shape that does not fly well in the atmosphere.
Despite the apparent limitations of space planes, they will probably benefit substantially from breakthroughs in propulsion and materials science. The Boeing X-37 is a small unmanned space plane being operated by the US Air Force. A proposal for combining a form of space plane with a tether system for orbital transfer is mentioned in the section below on tether systems. It will be interesting to see whether space planes are ever developed for human transport into orbit.
Mass Driver TRL 4
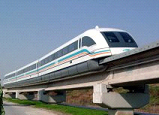
A mass driver, also called an electromagnetic catapult, uses non-propulsive methods to get a vehicle up to speed. It would likely resemble a magnetically-levitating train whose track curved upward into the sky. Even if only half a vehicle’s final velocity could be delivered by the mass driver, it would cut the required rocket fuel down by much more than half. Most of a traditional rocket’s fuel is used at the beginning of flight to push other stored fuel; as soon as it is lighter, a small amount of fuel can deliver a much larger acceleration. A vehicle that carried humans and cargo into space from a mass driver would probably require some amount of rocket power for the later part of the trip.
An additional advantage of a mass driver is that the heaviest air resistance would be at the bottom of the track. A vehicle would not need to use fuel to fight this air resistance. The summit of Mt. Everest has an air pressure of only one third that of sea level. It might be optimal, therefore, to build such a structure up the side of a tall mountain. If a vehicle was sent up the west side of a mountain, it could also take advantage of the Earth’s eastward rotation to get a boost in velocity. A mass driver would certainly be a colossal project, but it would require no major technological breakthroughs.
Space Elevator TRL 3
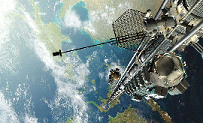
The space elevator concept has been around for decades. Rather than building the structure upwards, it actually hangs from geostationary orbit. An object in geostationary orbit always remains above the same point on Earth’s equator-if a space elevator had its center of mass positioned here, it would need no structural support from Earth.
A cable would then hang down to the surface. Another cable would “hang” out into space as a counterweight to keep the center of mass in geostationary orbit. Some kind of vehicle, often called a climber, would then carry payloads up the cable to the desired height or velocity. The space elevator has also been called a “skyhook” because of how it hangs down from space.
Major technological challenges for the space elevator persist, specifically finding a material strong and light enough to use as a cable. The cable would need to be more than 22,000 miles (35,000 kilometers) long, which is considerably longer than the diameter of Earth itself. Supporting its own weight would be the primary difficulty. Advances in nanotechnology, particularly in carbon nanotubes, may prove promising for space elevators. Other challenges include terrestrial weather hazards, impacts with meteoroids and orbiting objects, and repair options.
Tether Systems TRL 2
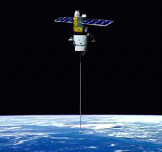
Tether propulsion involves transferring momentum between two spacecraft. As a method of non-rocket space launch, a tether system could help boost a space plane from a suborbital trajectory to a full orbital flight path. For this to work, a spinning tether station must be in an orbit that allows it to dip to a very low altitude-near the lower boundary of space.
Such an altitude, however, would require it to move very fast compared to aircraft speeds. To allow for a rendezvous between space plane and station, a very fast plane must be used. The tether station also must extend one or more tethers outward and spin to reduce the velocity of the tether tips at low altitude. Though the station would be orbiting, it would appear as if it were “rolling” on the top of the atmosphere, with the bottom position of the tethers moving much slower than the top. The slower-moving ends of tethers at low altitudes would allow fast-moving space planes to latch on.
Tether systems, which require tethers kilometers in length, generally must face the same risks of impact with orbiting objects as the space elevator does. There is the additional challenge of getting the space plane in position at the right time, latching on, and unlatching at the right time. Though no tether propulsion system has yet been tested, these concepts are gaining more attention in recent times. Prospects for tether systems will benefit from advances in materials science and aeronautics research.
Flow of Resources
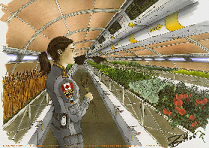
A variety of technologies are being pursued to help reuse and recycle resources in space. Astronauts aboard the International Space Station (ISS) already reuse water, which in the end is much more pure than public water in a typical developed city. Plants can allow for the exchange of carbon dioxide for oxygen, and can eventually provide food matter by using waste as fertilizer. Packaging of goods will be made to be as recyclable as possible out of practical necessity. This process of creating an artificial ecosystem is commonly referred to as “closing the loop”, and will minimize the need for new resources.
The process of obtaining resources from an extraterrestrial environment-such as the Moon, Mars, or an asteroid-is called in-situ resources utilization (ISRU). ISRU techniques may allow astronauts to produce breathing oxygen, drinking water, and rocket fuel from extraterrestrial matter. Water ice exists in permanently shadowed craters on the surface of the Moon.
On Mars, methane and oxygen can be created from atmospheric carbon dioxide as long as astronauts bring a supply of hydrogen. Methane can be used as a rocket fuel or to power internal combustion engines. It may be possible to construct functional solar panels from lunar soil. Finally, bricks could be worked from local materials to provide structural support and protect habitats from radiation. ISRU will allow easier access to needed resources.
Generating a flow of resources that does not depend on Earth is an essential step in creating self-sufficient space colonies. In the initial phase of space settlement, however, many hi-tech assets will have to be imported from Earth. Nuclear reactors in particular are extremely complicated machines, especially those intended for space applications. Nevertheless, space habitats will depend critically on such technology. When the most complicated of components can be manufactured outside the Earth’s atmosphere, space habitats will become truly self-sufficient.
Health Challenges
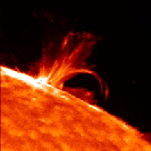
Living in space presents a number challenges to human health. These are typically related to radiation, gravity, or sanitation. Although much experience has been gained over the past decades of spaceflight, some key uncertainties remain. The fields of astronautical hygiene and space medicine have emerged to prevent and treat health complications in space.
Radiation coming from both the Sun and our galaxy can be dangerous to astronaut health. Many of these particles are deflected away from humans on the surface by Earth’s magnetic field; the radiation environment in space, however, is very different. Solar radiation is especially dangerous only during peak events.
To alert astronauts of these dangerous episodes of space weather, robotic spacecraft could be used as part of an interplanetary warning system. During such events, astronauts could retreat to solar “storm shelters.” Galactic cosmic rays, on the other hand, cannot generally be forecast as well as solar radiation. Countermeasures to mitigate this threat will have to be more passive and continuous.
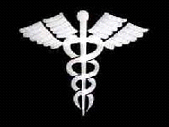
When in orbit, astronauts experience a negligible amount of gravity in their reference frame. This is not because the local gravitational field is weak; rather, it is because they are continuously accelerating, or falling, with gravity. The effect on the body, nevertheless, is the same as if there were no gravity. The absence of gravity tends to cause muscle atrophy and a reduction in bone density.
For this reason, astronauts on the ISS exercise for an hour or more per day. Around 45% of astronauts also report an initial bout of nausea and discomfort upon reaching space-this is commonly referred to as space sickness. While serious, the symptoms of space sickness have not been shown to last more than 72 hours. An effective countermeasure to these effects may be possible with artificial gravity, which can be generated by spinning a space habitat. There is no doubt that artificial gravity can be created, but it has not yet been put into practice; therefore, unforeseen health challenges may be involved with artificial gravity.
One of the key weaknesses of the three-person Apollo spacecraft was its waste management system. Urination was possible through attachable “relief tubes”, which dumped urine into space. Bags were used to store fecal matter. Besides being extremely frustrating to use, this system would not be sanitary for longer periods of time. Waste management is one area that has significantly improved on space stations since the Apollo era.
Space toilets have been used on the Space Shuttle and ISS. However, there is still much room for improvement in the design, redundancy, and placement/integration of space toilets in space habitats. Likewise, procedures to maintain sanitation in food storage and trash disposal will need to be continually improved. Finally, self-sufficient space habitats will require methods of preventing the spread of contagious illnesses.
Ark I
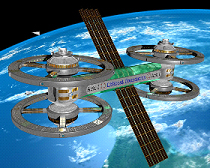
Ark I is a self-sustaining space habitat being designed to ensure the survival of humanity in the event Earth becomes uninhabitable. It is intended to incorporate several ambitious technologies and techniques to achieve long-term sustainability. Artificial gravity will be created by spinning two sets of wheels, which form the living quarters.
A complex ecosystem will be created by bringing plant and animal life into space. Resources from the Moon and asteroids will be used to reduce the habitat’s dependence on Earth. Ark I will be powered by nuclear fission reactors and solar panels. Finally, to guard against the further spread of any damage, individual modules of the habitat will be capable of sealing themselves off from the rest of the spacecraft.
Ark I is significant to the establishment of space habitats because it is one of the first attempts to create a self-sufficient environment and ecosystem away from the Earth. The project will serve as an impetus to improve methods of accessing space. It will help to develop new ways of managing and obtaining resources. It will also serve as a test bed for advances in astronautical hygiene and space medicine.