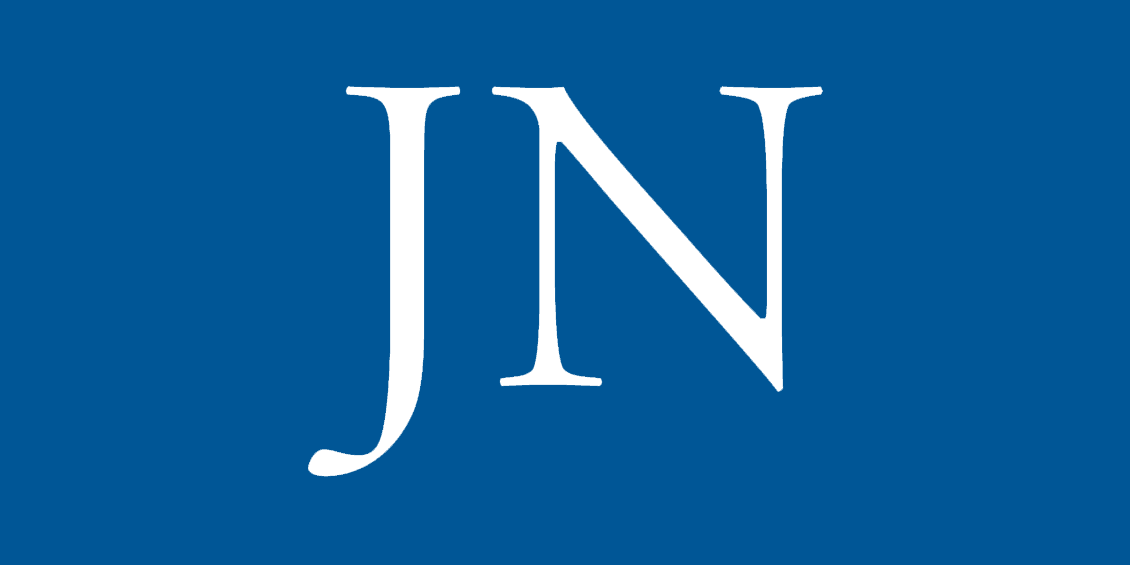
Lucid dreaming (LD) is a state of conscious awareness of the ongoing oneiric state, predominantly linked to REM sleep. Progress in understanding its neurobiological basis has been hindered by small sample sizes, diverse EEG setups, and artifacts like saccadic eye movements. To address these challenges in the characterization of the electrophysiological correlates of LD, we introduced an adaptive multi-stage preprocessing pipeline, applied to human data (male and female) pooled across laboratories, allowing us to explore sensor-and source-level markers of LD. We observed that, while sensor-level differences between LD and non-lucid REM sleep were minimal, mixed-frequency analysis revealed broad low-alpha to gamma power reductions during LD compared to wakefulness. Source-level analyses showed significant beta power (12−30 Hz) reductions in right central and parietal areas, including the temporo-parietal junction, during LD. Moreover, functional connectivity in the alpha band (8−12 Hz) increased during LD compared to non-lucid REM sleep. During initial LD eye signaling compared to baseline, source-level gamma1 power (30−36 Hz) increased in right temporo-occipital regions, including the right precuneus. Finally, functional connectivity analysis revealed increased inter-hemispheric and inter-regional gamma1 connectivity during LD, reflecting widespread network engagement. These results suggest that distinct source-level power and connectivity patterns characterize the dynamic neural processes underlying LD, including shifts in network communication and regional activation that may underlie the specific changes in perception, memory processing, self-awareness, and cognitive control. Taken together, these findings illuminate the electrophysiological correlates of LD, laying the groundwork for decoding the mechanisms of this intriguing state of consciousness.
Significance statement Lucid dreaming (LD) is a unique state of oneiric awareness, where individuals recognize they are dreaming while still in the dream. LD neural correlates remain elusive, as it is very rare and difficult to reproduce in the laboratory. Using an advanced preprocessing pipeline, we harmonized diverse EEG datasets to analyze the largest LD sample to date. We observed gamma power increases in the precuneus during initial eye lucidity signaling, beta power reductions in parietal areas, including the temporo-parietal junction, and enhanced alpha and gamma connectivity during LD over non-lucid REM sleep. These findings shed light on how the brain generates self-referential awareness and volitional action even during sleep.