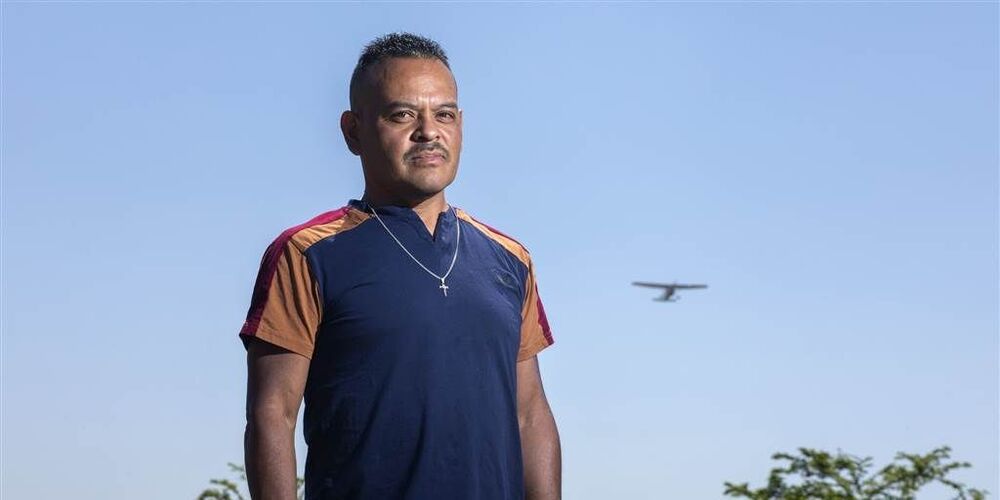
While leaded gasoline was fully phased out in 1996 with the passage of the Clean Air Act, it still fuels a fleet of 170000 piston-engine airplanes and helicopters. Leaded aviation fuel, or avgas, now makes up “the largest remaining aggregate source of lead emissions to air in the U.S.,” according to the Environmental Protection Agency.
Meanwhile residents continue to live with the air quality that comes with living near an airport where small planes burning leaded fuel fly in and out, said Alarcon, who is also a volunteer organizer with the nonprofit tenant advocacy group Vecinos Activos. It’s also unclear to air quality experts and residents what is arguably safe.
“There is no bright line that says ‘Above this concentration lead is safe and below this concentration’ that it is not. You’d have to make a policy decision,” said Jay Turner, an engineering education professor at Washington University in St. Louis and member of the EPA’s Science Advisory Board. “We’re really careful to come back to this point that just because public areas might meet the EPA standard [for lead] doesn’t mean zero risk or zero concern.”
Piston-engine airplanes have been a mainstay in aviation since they were first introduced in the early 20th century, according to Walter Desrosier, vice president of engineering and maintenance with the aviation industry group General Aviation Manufacturers Association. Since World War II, piston engines have been widely used by pilot hobbyists, aviation students and government agencies because of their high-performing engines and reliability to stay aloft amid rapid changes in temperature, pressure and altitude. They also cost less at $400000 to $500000.